News
techArt 4: Beyond Materials
Die Projektreihe Kunst und Wissenschaft im Dialog bringt zum vierten Mal kreative Köpfe aus beiden Welten zusammen. Vom 14. bis 16. März lädt die TU Wien zur Ausstellung mit 50 Originalwerken von Asya Marakulina und Chien-Yu Lin in den Festsaal ein. Inspiriert von der antiken Vier-Elemente-Lehre und aktuellen wissenschaftlichen Konzepten, entsteht ein spannender Dialog über Materialien, Umwelt und Wandel.
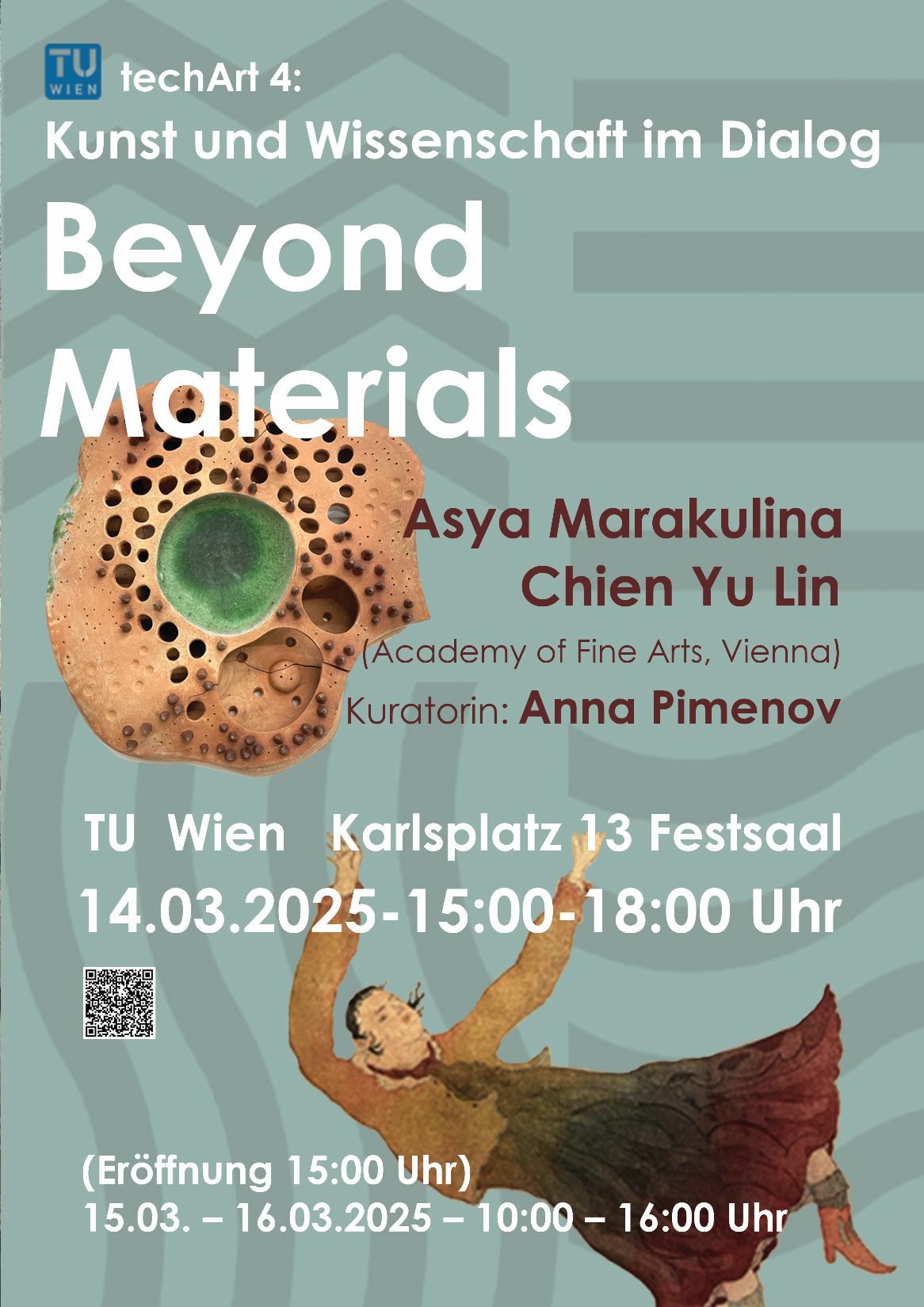
We welcome Xin Liu
Xin got his PhD in 2020 from Beijing Normal University, China. During his Ph.D study, he mainly focused on exploring emergent quantum phenomena in oxide heterostructures and superlattices of strongly correlated electron system, especially for 5d iridates with strong spin orbit coupling. He did his first postdoc at SiwssFEL Bernina group in PSI, Switzerland, where he studied the magnetic and electronic properties in strongly correlated oxides by using ultrafast techniques (e.g. optical pump probe and time-resolved XRD) and synchrotron-based techniques. Xin now join the solid-state spectroscopy group as a postdoctoral researcher, working in the field of terahertz spectroscopy on strongly correlated materials.
Successful PhD Thesis Defense
Congratulations to our group member and friend Janek Wettstein for successfully defending his PhD thesis, "Novel phenomena in multiferroic rare-earth metal oxides", supervised by Prof. Dr. A. Pimenov and Dr. Dávid Szaller.
Holographische Botschaft codiert in einfachem Plastik
Wichtige Daten, etwa eine Bitcoin-Wallet-Adresse, lassen sich mit 3D-Drucker und Terahertz-Strahlen recht einfach in gewöhnliches Plastik einspeichern und verbergen, zeigt die TU Wien.
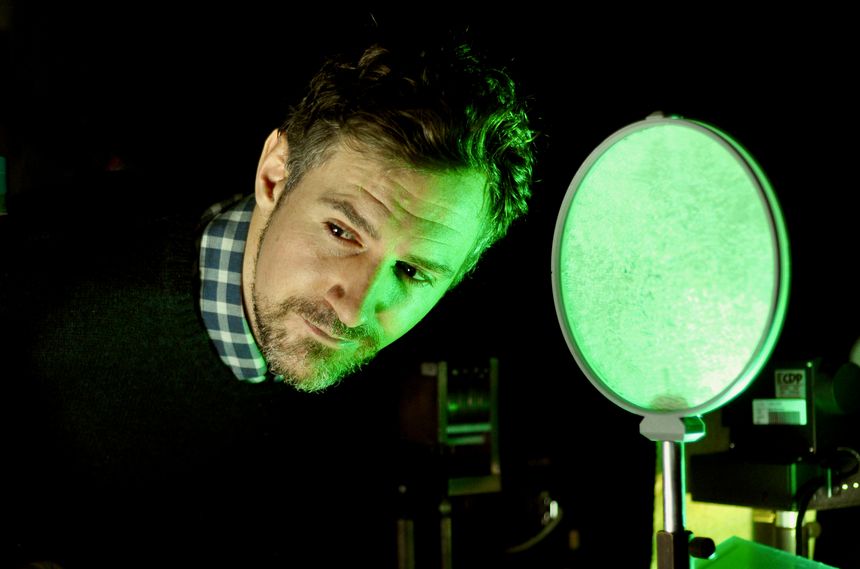
Es gibt viele Möglichkeiten, Daten abzuspeichern – etwa digital, auf einer Festplatte, oder aber auch analog, zum Beispiel in einem Hologramm. Meist ist es technisch ziemlich kompliziert, Hologramme herzustellen: Man verwendet dafür normalerweise hochpräzise Lasertechnologie.
Wenn es aber einfach nur darum geht, Daten in einem physischen Objekt abzuspeichern, dann funktioniert Holographie auch viel einfacher, wie man an der TU Wien nun zeigen konnte: Aus ganz normalem Plastik lässt sich im 3D-Drucker eine Blende herstellen, in der man zum Beispiel einen QR-Code abspeichern kann. Ausgelesen wird die Botschaft mit Terahertz-Strahlen – also mit elektromagnetischen Feldern, die für das menschliche Auge unsichtbar sind.
Das Hologramm als Datenspeicher
Ein Hologramm ist etwas völlig anderes als ein gewöhnliches Bild. Bei einem gewöhnlichen Bild hat jeder Bildpunkt eine klar definierte Position. Reißt man ein Stück ab, ist ein Teil des Inhalts verloren.
Beim Hologramm allerdings entsteht das Bild durch Beiträge aller Bereiche des Hologramms gleichzeitig. Nimmt man ein Stück des Hologramms weg, erzeugt der Rest immer noch das vollständige Bild (wenn vielleicht auch weniger scharf.) Beim Hologramm ist die Botschaft nicht punktweise gespeichert, sondern die gesamte Botschaft ist über das gesamte Hologramm verteilt.
„Wir haben dieses Prinzip auf Terahertzstrahlen angewendet“, sagt Evan Constable vom Institut für Festkörperphysik der TU Wien. „Das sind elektromagnetische Strahlen im Bereich von rund hundert bis zu mehreren tausend Gigahertz, also vergleichbar mit der Strahlung eines Mobiltelefons oder eines Mikrowellenofens – aber mit deutlich höherer Frequenz.“
Diese Terahertzstrahlung wird auf eine dünne Plastikblende geschickt. Diese Blende ist für die Terahertzstrahlen beinahe transparent, hat aber einen höheren Brechungsindex als die umgebende Luft, verändert die einfallende Welle also ein jeder Stelle ein bisschen anders. „Von jedem Punkt der Blende geht dann eine Welle aus, und all diese Wellen überlagern sich miteinander“, sagt Evan Constable. „Wenn man die Dicke der Blende Punkt für Punkt genau richtig angepasst hat, dann ergibt sich aus der Überlagerung all dieser Wellen genau das gewünschte Bild.“
Es ist so ähnlich als würde man auf ausgeklügelte Weise viele kleine Steinchen in einen Teich werfen, sodass sich die Wasserwellen all dieser Steinchen zu einem ganz bestimmten Gesamt-Wellenmuster addieren.
Ein Stück billiges Plastik als High-Tech-Speicher für Wertvolles
Auf diese Weise gelang es, eine Bitcoin-Wallet-Adresse (bestehend aus 256 bit) in eine Plastikblende zu codieren. Durchstrahlt man diese Blende mit Terahertz-Stahlen der richtigen Wellenlänge, entsteht ein Terahertz-Strahlenbild, das genau den gewünschten Code ergibt. „So kann man einen Wert von zehntausenden Euro in ein Objekt auf sichere Weise einspeichern, das nur ein paar Cent kostet“, sagt Evan Constable.
Damit die Blende den richtigen Code erzeugt, muss man zunächst exakt ausrechnen, an welchem Punkt sie welche Dicke haben muss, damit sie die Terahertzwelle genau auf die richtige Weise verändert. Den Code, mit dem man dieses Dickeprofil erhält, stellten Evan Constable und seine Mitarbeiter gratis auf Github zur Verfügung. „Dann braucht man nur noch einen ganz gewöhnlichen 3D-Drucker, um die Blende auszudrucken, und schon hat man die gewünschte Information holographisch gespeichert“, erklärt Constable. Ziel der Forschungsarbeit war es nicht nur, Holographie mit Terahertzwellen möglich zu machen, sondern auch zu demonstrieren, wie gut die Technik rund um diese Wellen fortgeschritten ist, und wie präzise sich dieser bisher noch eher ungewöhnliche Bereich der elektromagnetischen Strahlung heute bereits technisch nutzen lässt.
Drei ESPRIT-Förderungen für die TU Wien
Mit ESPRIT – kurz für „Early-Stage-Programme: Research–Innovation–Training” – fördert der FWF hochqualifizierte PostDocs über die Dauer von drei Jahren. Neben dem Gehalt werden projektspezifische Kosten von bis zu 75.000 EUR von der Förderung gedeckt.
Maksim Savchenko und sein Projekt „Polarisationsproblem und neue Effekte bei THz-Absorption“
Maksim Savchenko arbeitet als PostDoc im Forschungsbereich Solid State Spectroscopy der TU Wien und untersucht das optische sowie das Transportverhalten von Halbleiterstrukturen unter Einwirkung von Gigaherthttps://www.nature.com/articles/s41598-024-56113-2z- und Terrahertz-Strahlung. Im Rahmen seines ESPRIT-Projekts plant er, das Problem der Polarisationsunempfindlichkeit der Zyklotronresonanz-Zyklotronabsorption zu lösen und die optischen Gegenspieler der Quantenoszillationen des Gleichstromtransports zu finden und zu untersuchen.
Der erste Teil des Projekts bezieht sich auf das kürzlich entdeckte Problem, dass die Zyklotronabsorption der Terrahertz-Strahlung unempfindlich gegenüber ihrer Polarisation ist. Unabhängig davon, ob die Lichtpolarisation linear oder zirkular ist, absorbiert die Struktur das Licht, als ob die Polarisation linear wäre. Dieses Verhalten widerspricht sehr grundlegenden und allgemein akzeptierten Erkenntnissen über die Wechselwirkung zwischen Licht und Materie in heterogenen Halbleiterstrukturen (üblicherweise als Drude-Theorie bezeichnet). Es wirft daher neue Fragen auf und erfordert die detaillierte Untersuchung des Phänomens.
Maksim Savchenko promovierte 2019 an der Novosibirsk Universität (Russland), wo er sich hauptsächlich mit Quantentransport und Magnetooptik von Quecksilber-Tellur-basierten topologischen Isolatoren beschäftigte. Sein erstes Postdoktorat absolvierte er 2021 an der Purdue Universität (USA), wo er lernte, wie Halbleiter in Reinraumanlagen hergestellt werden.
Wir gratulieren herzlich zu diesen Erfolgen!
techArt
17, 18, 19 November präsentiert die Projektreihe techArt: Kunst und Wissenschaft im Dialog
Alte Kunst - Neue Wissenschaft
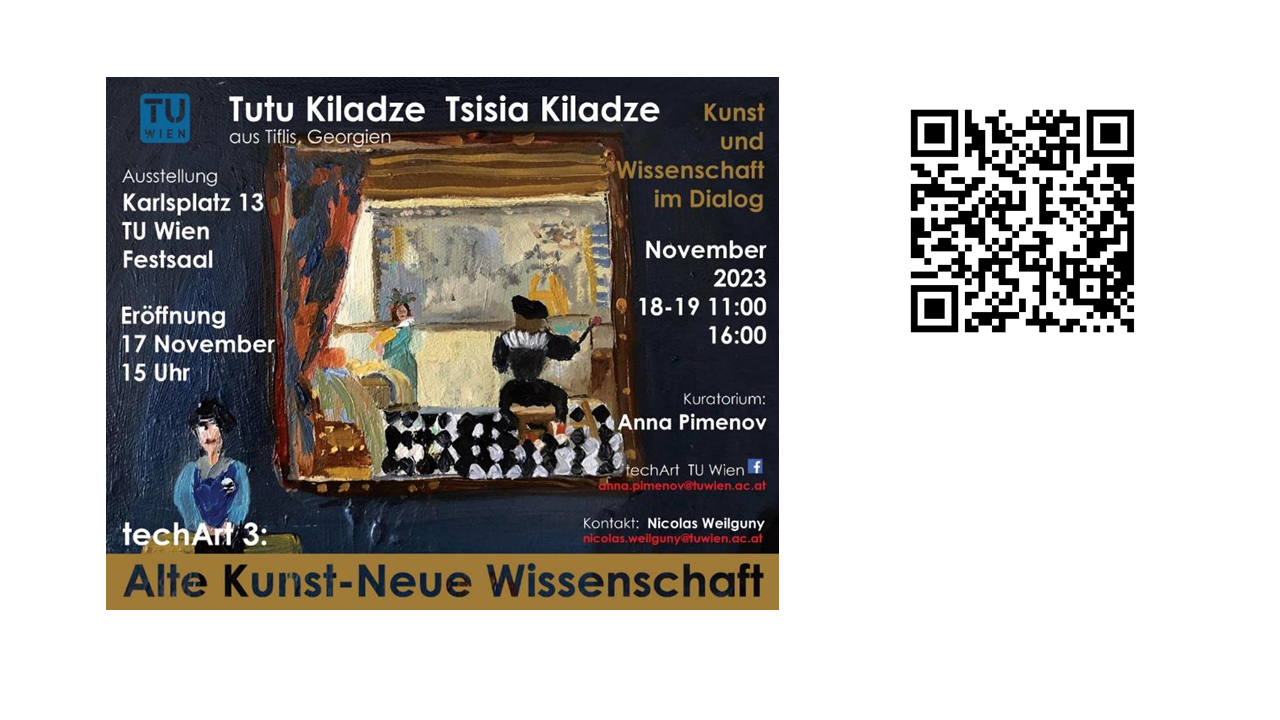
LEES 2023
Happy to announce the dates for the conference Low-Energy Electrodynamics in Solids 2023 that our group is organizing. It takes place in Sankt Pölten, Austria, 25.-30.06.2023.
The fine structure constant measured directly
Forschende der TU Wien und der University of California Los Angeles haben eine neue Methode entwickelt, um die Feinstrukturkonstante direkt zu bestimmen. Sie beobachteten die schlagartige Änderung der Polarisationsrichtung eines linear polarisierten Laserstrahls beim Durchqueren einer nm-dicken Schicht (Cr0.12Bi0.26Sb0.62)2Te3. Die Richtung ändert sich in festen Winkelschritten, die von der Feinstrukturkonstante abhängen, sodass sich diese direkt ablesen lässt.
Physik Journal Nr.1 2023/ Deutschland
A Quantum of an Angle
The fine structure constant is one of the most important natural constants of all. At TU Wien, a remarkable way of measuring it has been found – it shows up as a rotation angle.
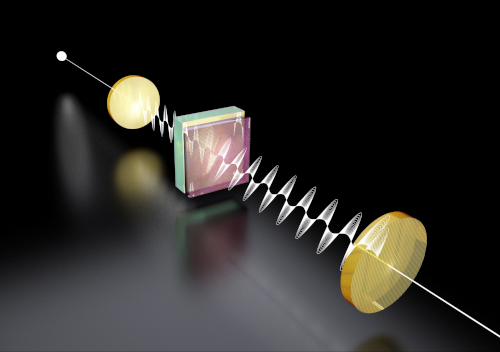
One over 137 - this is one of the most important numbers in physics. It is the approximate value of the so-called fine structure constant - a physical quantity that is of outstanding importance in atomic and particle physics.
There are many ways to measure the fine structure constant - usually it is measured indirectly, by measuring other physical quantities and using them to calculate the fine structure constant. At TU Wien, however, an experiment has now been performed, in which the fine structure constant itself can be directly measured - as an angle.
1/137 - the secret code of the universe
The fine structure constant describes the strength of the electromagnetic interaction. It indicates how strongly charged particles such as electrons react to electromagnetic fields. If the fine structure constant had a different value, our universe would look completely different - atoms would have a different size, so all chemistry would work differently, and nuclear fusion in the stars would be completely different as well.
A much-discussed question is whether the fine structure constant is actually constant, or whether it could possibly have changed its value a little over billions of years.
Direct measurements instead of calculations
"Most important physical constants have a specific unit - for example, the speed of light, which can be given in the unit of meters per second," says Prof. Andrei Pimenov from the Institute of Solid State Physics at TU Wien. "It's different with the fine structure constant. It has no unit, it is simply a number – it is dimensionless."
But usually, when the fine structure is measured, various quantities with different physical units have to be measured, and then the value of the fine structure constant is inferred from these results. "In our experiment, on the other hand, the fine structure constant itself becomes directly visible," says Andrei Pimenov.
A thin film that rotates the light
A laser beam is polarised linearly - the light oscillates exactly in vertical direction. Then the beam hits a layer of a special material that is only a few nanometres thick. This material has the property of changing the polarisation direction of the light. "’A material rotating the polarisation of a laser beam is, by itself, nothing unusual. Different materials can do this; the thicker the material layer, the more the polarisation of the laser is rotated. But we are dealing with a completely different effect here," explains Andrei Pimenov. "In our case, the polarisation is not rotated continuously - it jumps."
When passing through the thin film, the polarisation direction of the light performs a quantum jump. After passing through, the light wave oscillates in a different direction than before. And when the size of this jump is calculated, an astonishing result appears: the quantum of this angular change is exactly the fine structure constant.
"We thus have direct access to something quite unusual: a quantum of rotation," says Andrei Pimenov. "The fine structure constant becomes immediately visible as an angle."
Wir gratulieren Dr.rer.nat. Jan Gospodaric zum Dissertationspreis der Stadt Wien
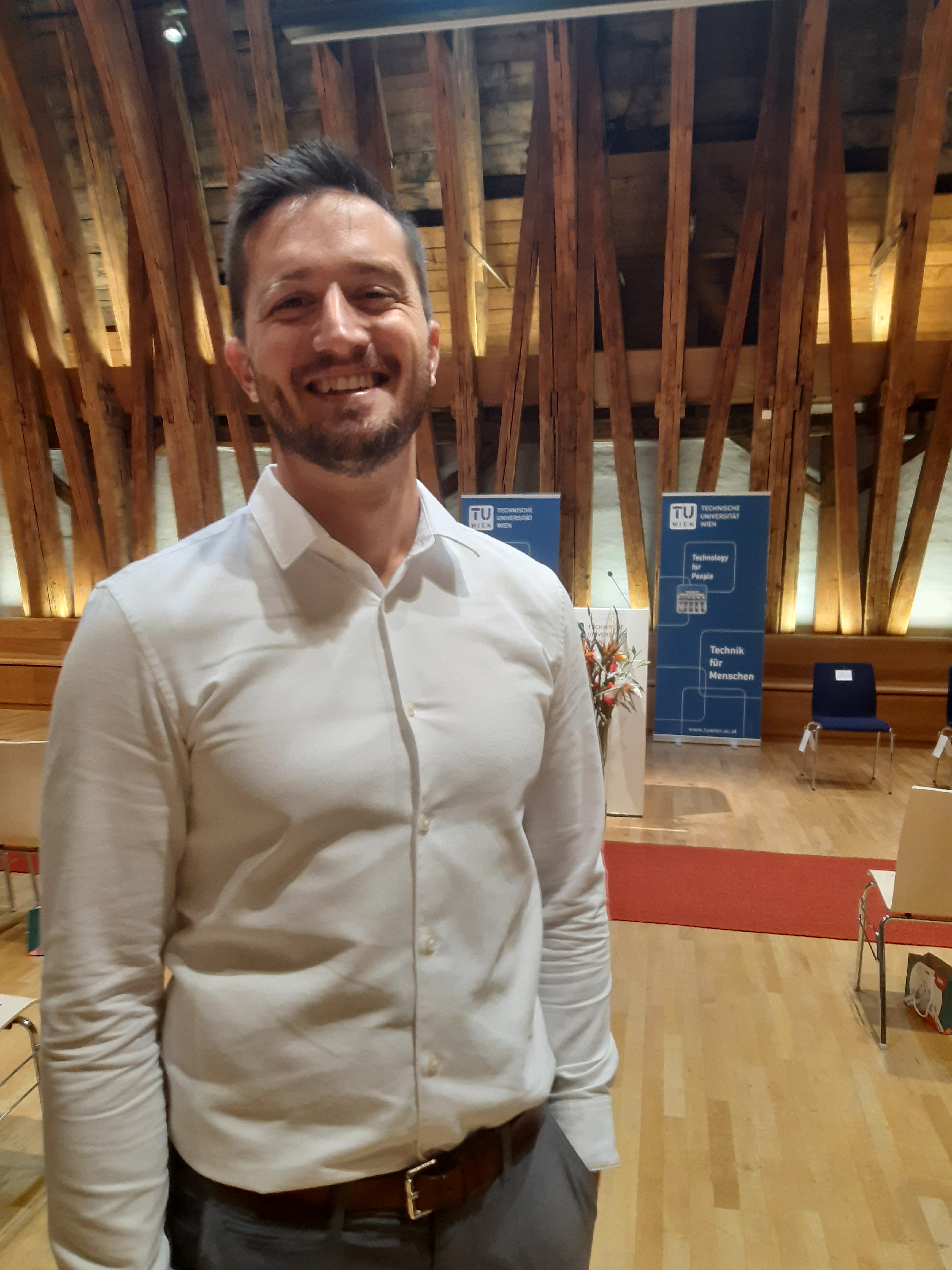
We welcome Maxim Ryzhkov
Maxim has attained his master's and bachelor's degree at Novosibirsk State University where he studied physics from 2016 to 2022. From 2018 until 2022, he performed scientific work in the Low-dimensional Electron Systems Group of Prof. Kvon. His bachelor thesis was devoted to the transport properties study of Dirac fermions in CdHgTe quantum wells. During master's studies, he focused on topological insulator state in CdHgTe quantum wells and won regional competition of fundamental research project for young scientists, then completed it successfully. The title of his master's thesis is "Transport Response of Quantum Well Based on the CdHgTe Supercritical Width". In September 2022 Maxim joined the group as a doctoral candidate.
We welcome Daniiar Khudaiberdiev
Daniiar studied physics at Novosibirsk State University in Russia from 2016 to 2022 where he performed his master thesis in the Low-dimensional Electron Systems Group of Prof. Kvon. In his thesis he investigated the two-dimensional topological Anderson insulator state realized on a disordered HgTe quantum well. In August 2022 Daniiar joined the group as a doctoral candidate.
Ein Viertaktmotor für Atome
Was man ein und wieder ausschaltet, ist normalerweise wieder im Ausgangszustand. Ein neues magnetisches Material muss dafür aber viermal geschaltet werden. Der Spin von Atomen bewegt sich dabei einmal im Kreis.
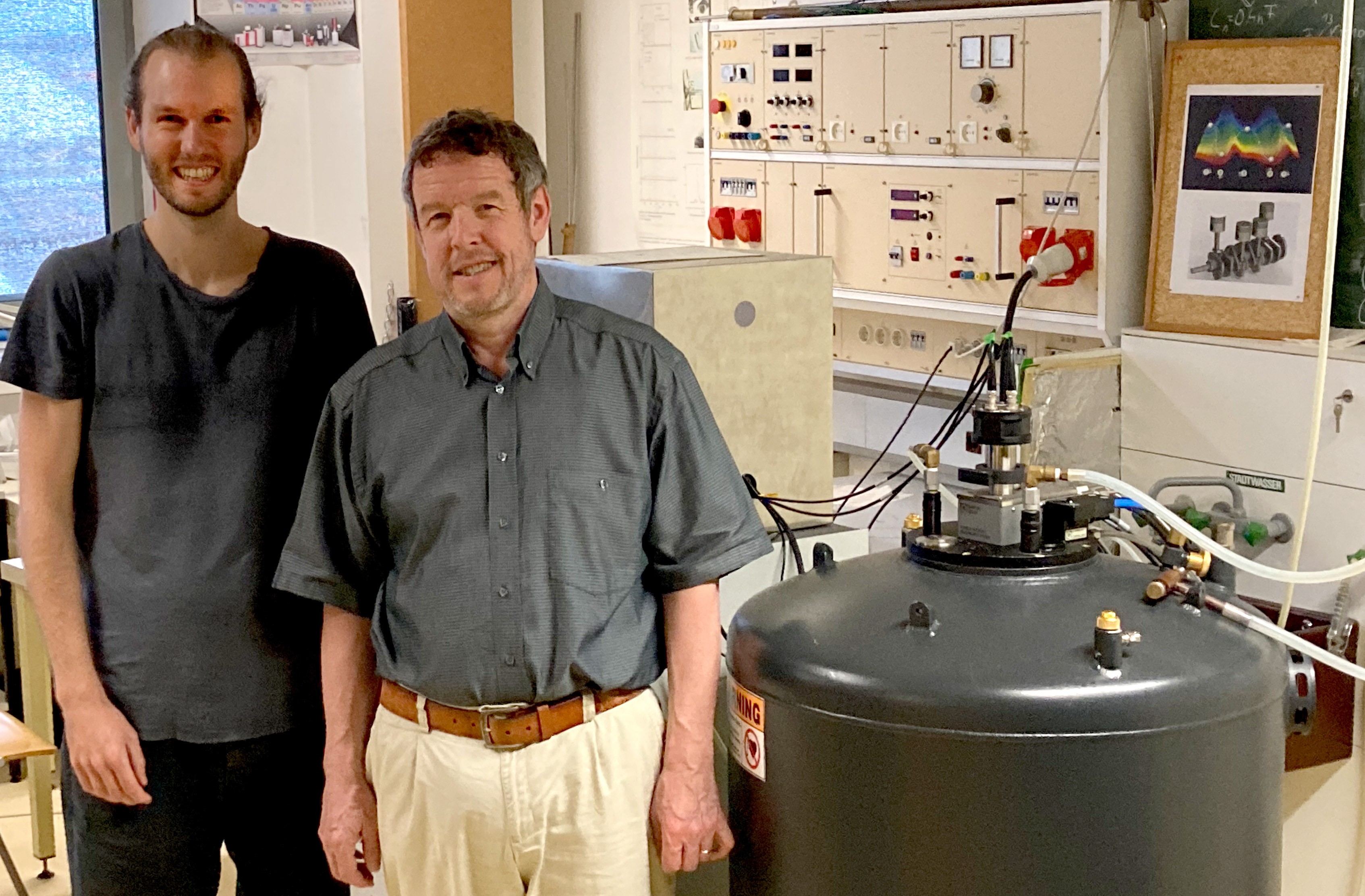
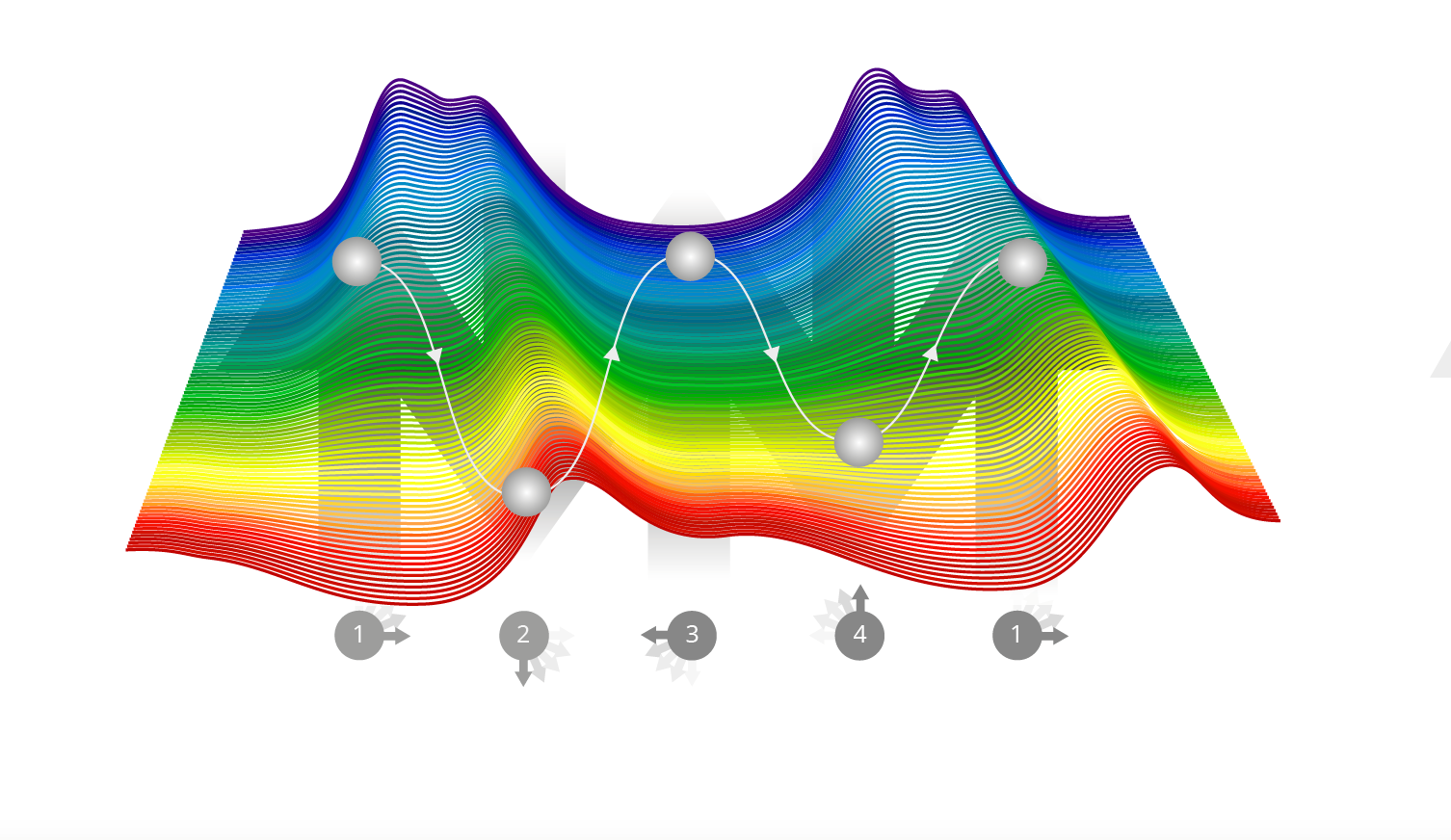
Wenn man ein Bit im Speicher eines Computers umschaltet und dann wieder zurückschaltet, dann hat man den Ursprungszustand wiederhergestellt. Es gibt nur zwei Zustände, die man „0 und 1“ nennen kann. An der TU Wien wurde allerdings nun ein verblüffender Effekt entdeckt: In einem Kristall basierend auf Oxiden von Gadolinium und Mangan stieß man auf einen atomaren Schalter, den man nicht bloß einmal, sondern zweimal hin und her schalten muss, bis der Ursprungszustand wieder erreicht ist. Der Spin von Gadolinium-Atomen wird während dieses doppelten Ein- und Ausschaltvorgangs insgesamt einmal im Kreis gedreht. Das erinnert an eine Kurbelwelle, bei der eine Auf-und-ab-Bewegung in eine Kreisbewegung umgewandelt wird. Dieses neue Phänomen eröffnet interessante Möglichkeiten in der Materialphysik, sogar Information könnte man mit solchen Systemen speichern. Im Fachjournal „Nature“ wurde der merkwürdige atomare Schalter nun präsentiert.
Kopplung von elektrischen und magnetischen Eigenschaften
Normalerweise unterscheidet man zwischen elektrischen und magnetischen Eigenschaften von Materialien. Elektrische Eigenschaften beruhen darauf, dass sich Ladungsträger bewegen – etwa Elektronen, die durch ein Metall wandern, oder Ionen, deren Position verschoben wird. Magnetische Eigenschaften hingegen hängen eng mit dem Spin von Atomen zusammen – dem Eigendrehimpuls des Teilchens, der in eine ganz bestimmte Richtung zeigen kann, ähnlich wie die Rotationsachse der Erde in eine ganz bestimmte Richtung weist. Allerdings gibt es auch Materialien, in denen elektrische und magnetische Phänomene sehr eng miteinander gekoppelt sind. An ihnen forscht Prof. Andrei Pimenov mit seinem Team am Institut für Festkörperphysik der TU Wien. „Wir haben ein spezielles Material aus Gadolinium, Mangan und Sauerstoff einem Magnetfeld ausgesetzt und gemessen, wie sich dabei seine elektrische Polarisierung verändert“, berichtet Andrei Pimenov. „Wir wollten also analysieren, wie sich die elektrischen Eigenschaften des Materials durch Magnetismus verändern lassen. Und überraschenderweise stießen wir dabei auf ein völlig unvorhergesehenes Verhalten.“
In vier Schritten zurück zum Anfang
Zu Beginn ist das Material elektrisch polarisiert – auf der einen Seite ist es positiv, auf der anderen Seite negativ geladen. Dann schaltet man ein starkes Magnetfeld ein – und an der Polarisation ändert sich nur wenig. Wenn man dann allerdings das Magnetfeld wieder ausschaltet, zeigt sich eine dramatische Änderung: Plötzlich kehrt sich die Polarisation um: Die Seite, die vorher positiv geladen war, ist danach negativ geladen, und umgekehrt. Nun kann man denselben Prozess ein zweites Mal durchlaufen: Wieder schaltet man das Magnetfeld ein, und die elektrische Polarisation bleibt ungefähr konstant. Wenn man das Magnetfeld ausschaltet, kehrt sich die Polarisation wieder um und erreicht somit wieder den ursprünglichen Zustand. „Das ist äußerst bemerkenswert“, sagt Andrei Pimenov. „Wir führen vier verschiedene Schritte durch, jedes Mal ändert das Material seine inneren Eigenschaften, aber nur zweimal ändert sich die Polarisation, daher erreicht man den Anfangszustand erst nach dem vierten Schritt.“
Viertaktmotor für Gadolinium
Eine genauere Betrachtung zeigt, dass die Gadolinium-Atome für dieses Verhalten verantwortlich sind: Sie ändern bei jedem der vier Schritte ihre Spinrichtung, jedes Mal um 90 Grad. „Es ist in gewissem Sinn ein Viertaktmotor für Atome“, sagt Andrei Pimenov. „Auch beim Viertaktmotor braucht es vier Schritte, bis der Ausgangszustand wieder erreicht ist – und der Zylinder bewegt sich dabei zweimal rauf und runter. Bei uns bewegt sich das magnetische Feld zweimal rauf und runter, bevor der Ausgangszustand wiederhergestellt ist und der Spin der Gadoliniumatome wieder in die ursprüngliche Richtung zeigt.“ Theoretisch könnte man solche Materialien verwenden, um Information zu speichern: Ein System mit vier möglichen Zuständen hätte eine Speicherkapazität von zwei Bits pro Schalter, statt des üblichen einen Bit Information für „0“ oder „1“. Besonders interessant ist der Effekt aber auch für die Sensortechnik: Man könnte etwa auf diese Weise einen Zähler für magnetische Pulse herstellen. Wichtige neue Inputs liefert der Effekt für die theoretische Forschung: Es ist ein weiteres Beispiel für einen sogenannten „topologischen Effekt“, eine Klasse von Materialeffekten, die seit Jahren in der Festkörperphysik viel Aufmerksamkeit auf sich ziehen und die Entwicklung neuer Materialien ermöglichen sollen.
techArt unterstützt ukrainische Künstlerin
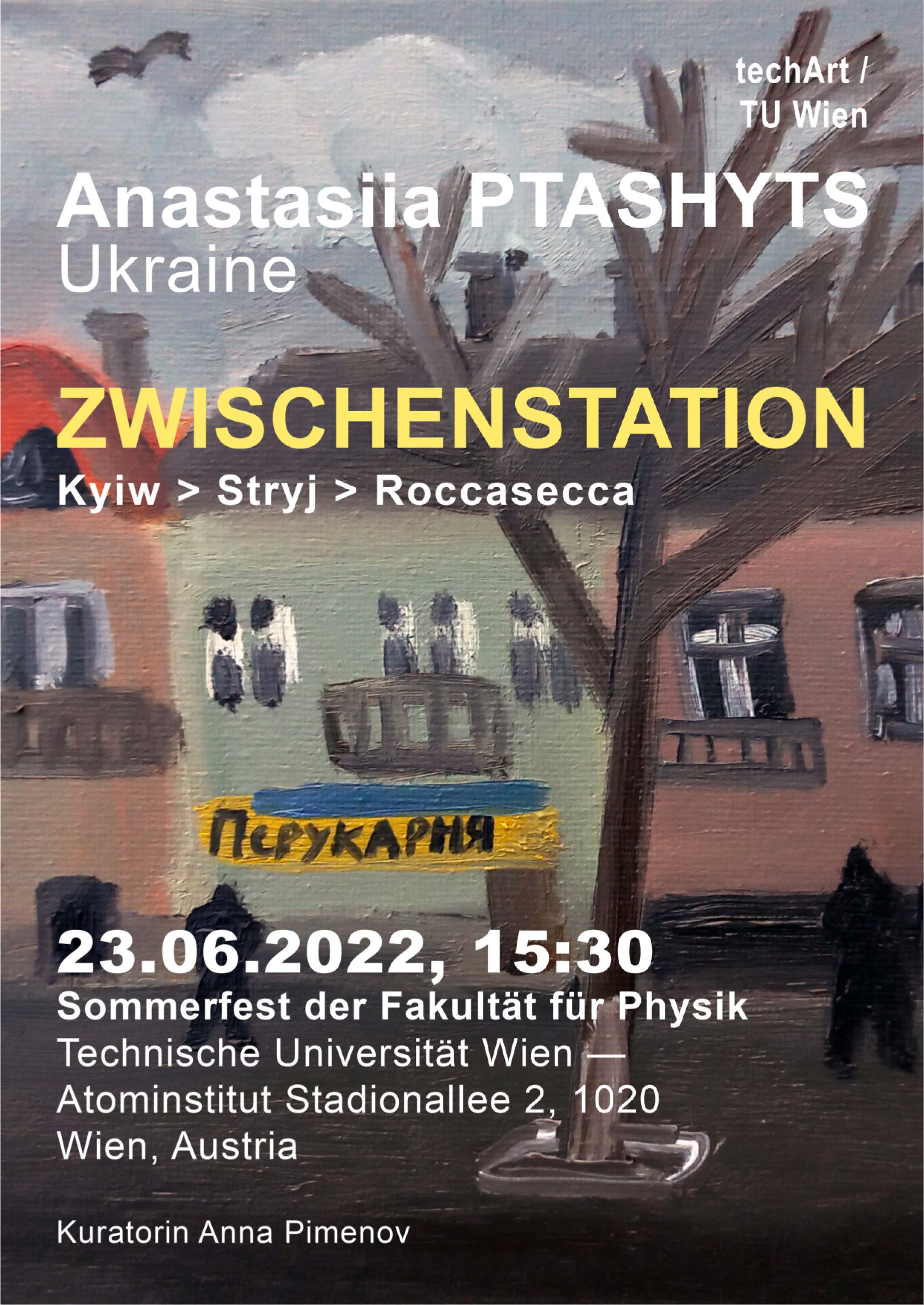
Successful PhD Thesis Defense
Congratulations to our group member and friend Lorenz Bergen for successfully defending his PhD thesis, "Far infrared spectroscopy of lattice dynamics in oxides with magnetically frustrated geometry", supervised by Prof. Dr. A. Pimenov and Dr. Evan Constable.
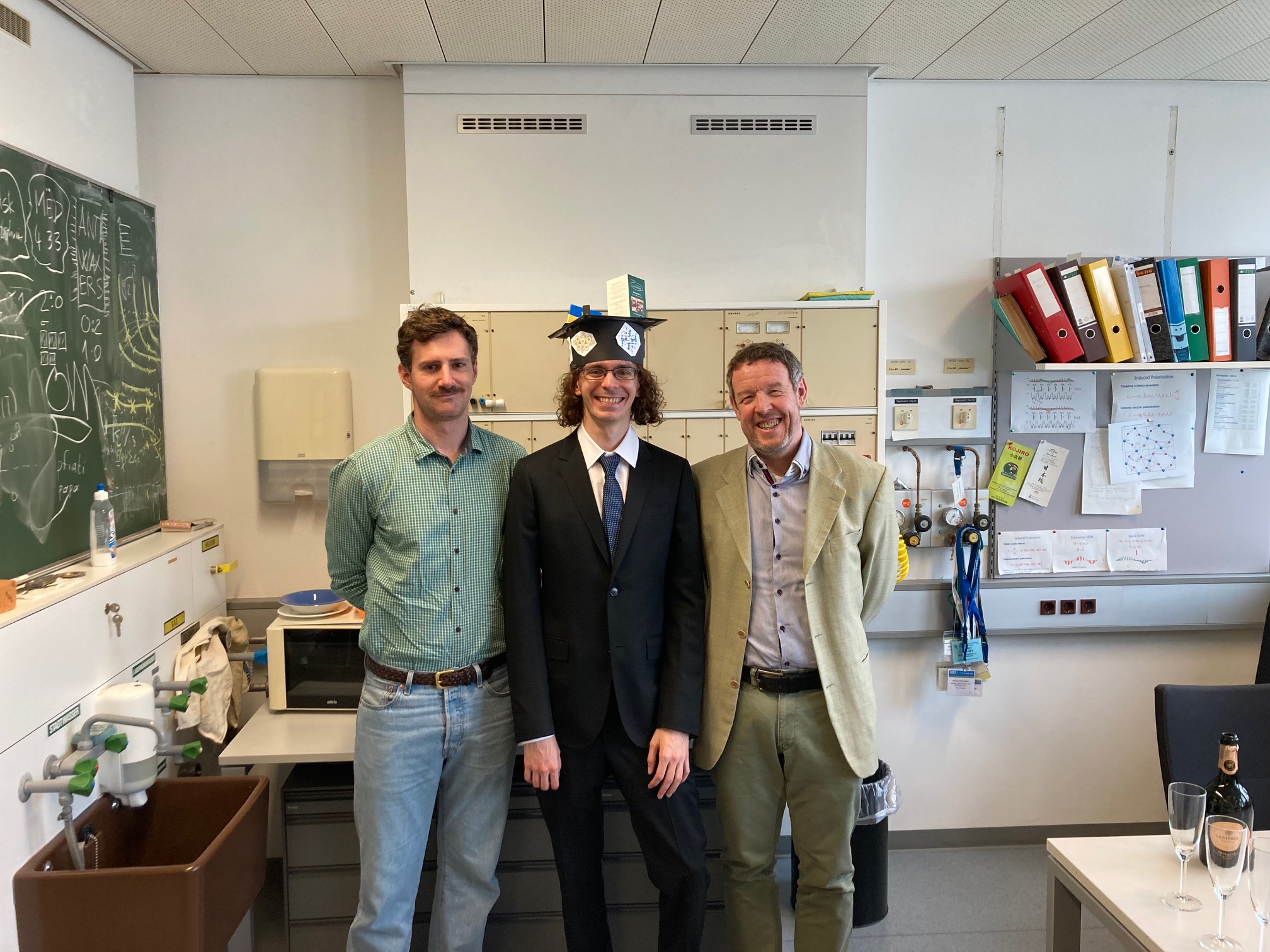
We welcome Maksim Savchenko
Maksim (Maxim) got his PhD at Novosibirsk State University/Rzhanov Institute of Semiconductor Physics in Russia in 2019, where he primarily worked on quantum transport and magnetooptic of HgTe-based topological insulators. He did his first PostDoc at Purdue University in USA in 2021 during which he mastered his fabrication skills in cleanroom facilities. Maksim now joins our group and plans to further study optic and transport response of semiconductor samples subject to GHz and THz radiation.
FWF – PhD positions
There is an opening for a doctoral position in our research group with a new FWF project. The aim of the project is the investigation and determination of the origin of giant magnetoelectric effects in new magnetic and multiferroic materials, using broadband dielectric (optical) spectroscopy. Applications within other research topics of the group are possible as well.
Highly qualified and motived national and international students are invited to apply.
Vienna is a fine location as regards culture and nature.
Please send an application to Andrei Pimenov
techArt
29, 30, 31 Oktober präsentiert die Projektreihe techArt: Kunst und Wissenschaft im Dialog
Karlsplatz anders
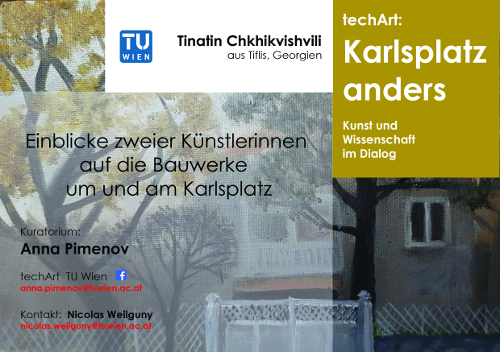
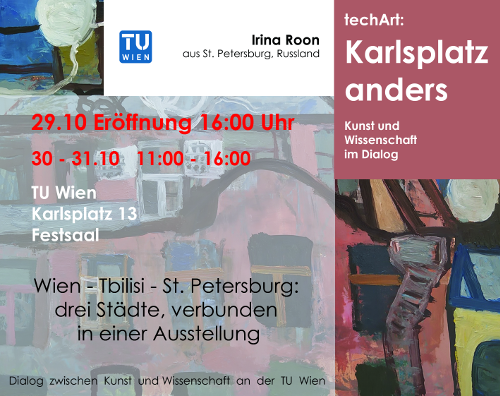
Successful online PhD Thesis Defense
Congratulations to our group member and friend Jan Gospodaric for successfully defending his PhD thesis online, "Magneto-optic study of novel HgTe-based thin films", supervised by Prof. Dr. A. Pimenov.
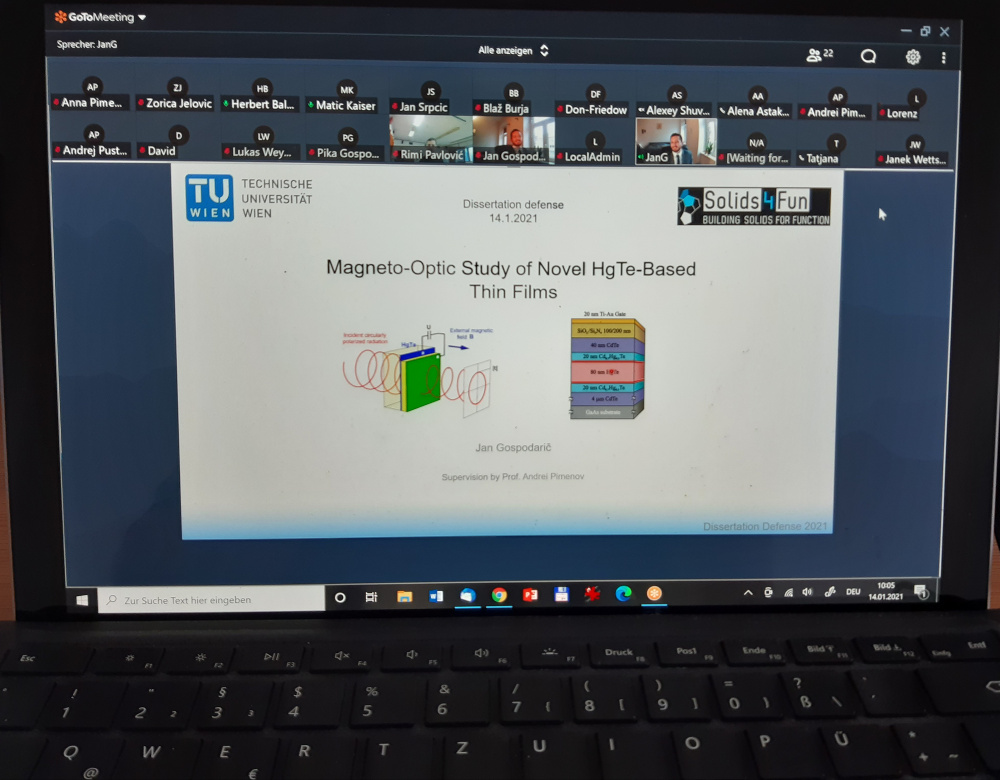
Successful online PhD Thesis Defense
Congratulations to our group member and friend Lukas Weymann for successfully defending his PhD thesis online, "Novel static and dynamic phenomena in magnetoelectric materials", supervised by Prof. Dr. A. Pimenov.
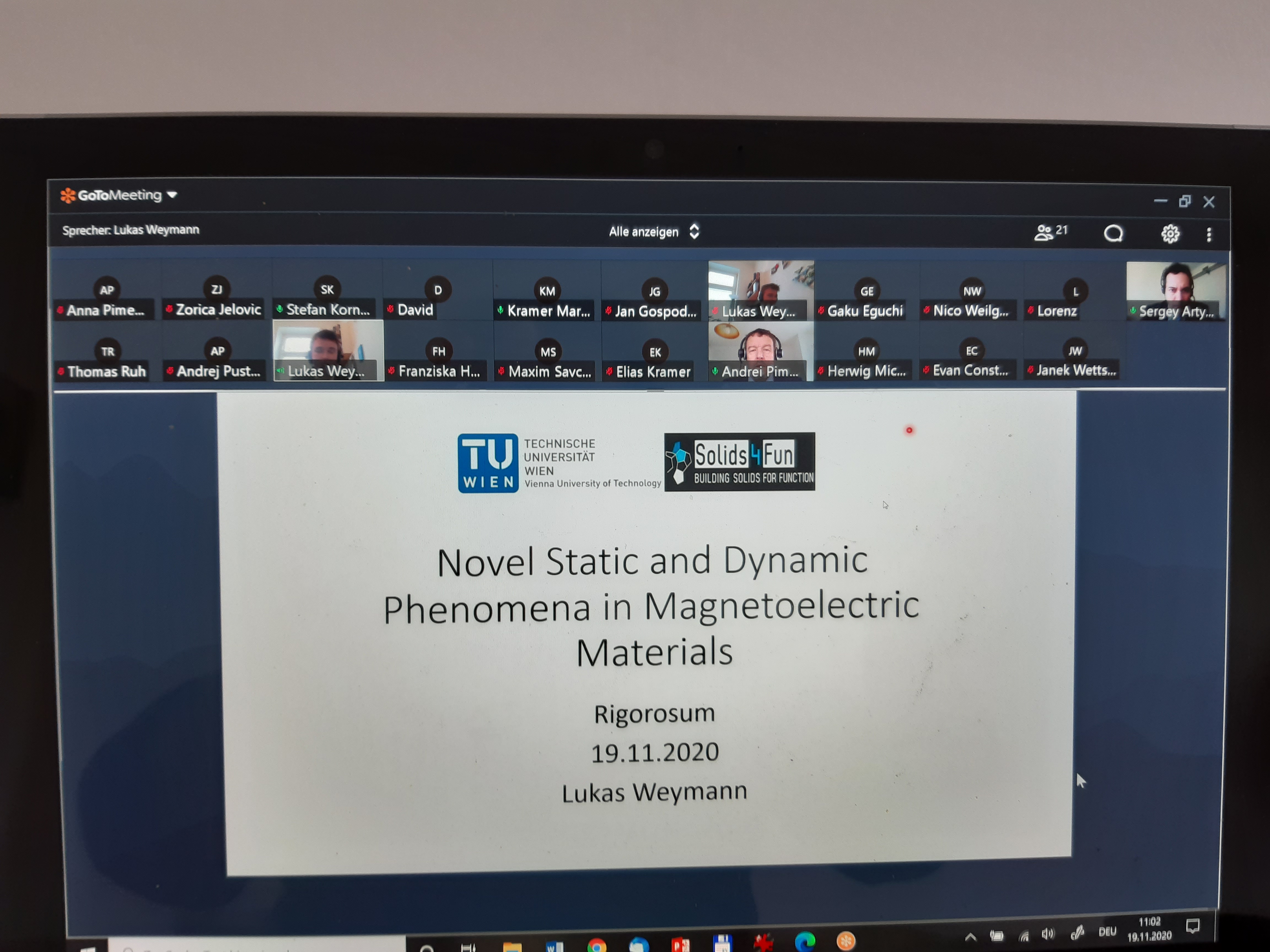
Wer wagt, gewinnt!
New Project: Quantum-optical phenomena in magnetoelectric crystals
Besonders kühne Forschungsideen wurden beim FWF-Wettbewerb „1000 Ideen“ gefördert – darunter gleich zwei von der TU Wien. Eine Mitarbeit an diesen Projekten ist im Rahmen von Postdoc-Stellen möglich!
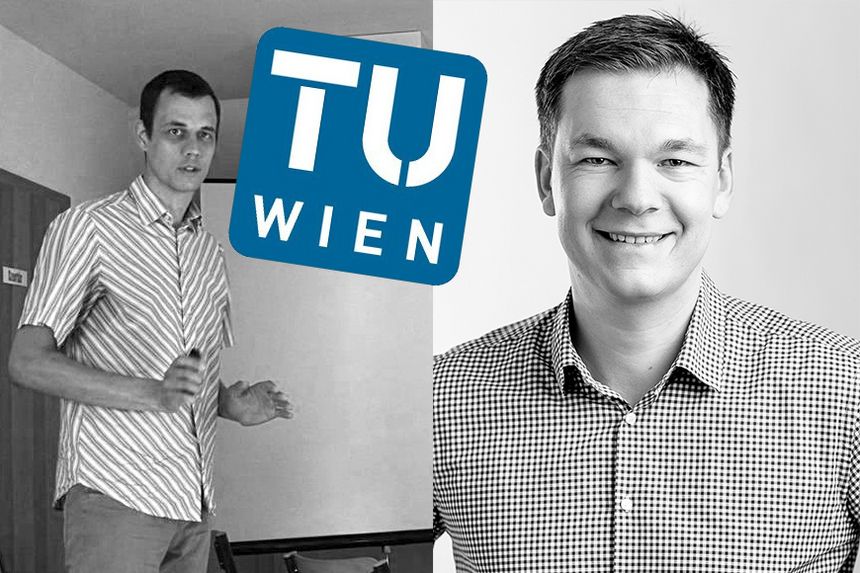
Es gibt Forschung, die auf einigermaßen vorhersagbare Weise Schritt für Schritt voranschreitet – und es gibt Forschung, die in Sprüngen verläuft, bei der originelle Ideen gefragt sind und ein gewisses Risiko eingegangen wird. Beides ist wichtig, doch bei der Ausschreibung „1000 Ideen“ des Wissenschafts-Förderungsfonds FWF, öffnet eine externe URL in einem neuen Fenster ging es um die zweite Sorte: Erstmals wurde explizit nach besonders gewagten Forschungsideen gesucht, die mit bis zu 150.000 Euro gefördert wurden.
Unter den Projekten, die in der ersten Ausschreibungsrunde den Zuschlag erhielten, sind zwei von der TU Wien: David Szaller vom Institut für Festkörperphysik möchte einen speziellen Quanteneffekt nutzen, um neuartiges Laserlicht zu erzeugen. Stefan Scheiner vom Institut für Mechanik der Werkstoffe und Strukturen möchte eine neue Herangehensweise entwickeln um das Wachstum von Bäumen vorherzusagen und Holz besser nutzbar zu machen. Die Projekte starten Anfang 2021, bereits jetzt kann man sich für neuausgeschriebene Stellen bewerben, die im Rahmen dieser Projekte geschaffen wurden.
Eine neue Sorte Licht
Laserstrahlen gehören längst zu unserem Alltag. In gewöhnlichen Festkörperlasern, wie wir sie etwa von Laserpointern kennen, wird die Lichtwelle zwischen zwei Spiegeln hin und her geschickt. Dabei gerät das Licht immer wieder in Kontakt mit den Atomen, die sich zwischen den Spiegeln befinden. Diese Atome senden weitere Photonen aus, die genau den Photonen entsprechen, die sich bereits im Laser befinden – so wird der Laserstrahl immer stärker. Die Atome im Laser wechselwirken nicht direkt miteinander, aber durch ihre Wechselwirkung mit dem Laserlicht benehmen sie sich alle exakt gleich und verstärken das Licht auf exakt identische Weise.
„Wenn sich der Abstand zwischen den beiden Spiegeln allerdings verändert, reagiert das Laserlicht darauf extrem empfindlich“, erklärt David Szaller. „Besser ist es, wenn nicht die Geometrie der Spiegel über das Laserlicht entscheidet, sondern ein kollektiver Quantenzustand des Materials. Das war bisher aber nur unter extrem großen Aufwand möglich, ganz nahe am absoluten Temperaturnullpunkt.“
An der TU Wien soll dieses Kunststück aber nun bei viel leichter zugänglichen Bedingungen möglich werden – indem man die magnetischen Momente von speziellen Atomen (seltenen Erden) nutzt. Wenn man Materialien verwendet, die eine ganz spezielle Kopplung von magnetischen und elektrischen Eigenschaften aufweisen, sollte es möglich sein, dadurch Laser-artige Strahlung mit extrem hoher Präzision zu erzeugen, und das bei gewöhnlichen Standardlabortemperaturen.
Das neue Mechanismus des magnetoelektrischem Effekts in Langasiten (Ho-LaGaSiO)
Polarisation in Holmium-dotierten Langasit wächst linear als Funktion des Magnetfeldes (siehe Bild) und ist gleichzeitig eine höchst nichtlineare Funktion der Magnetfeldrichtung (inset). Dieses Verhalten steht im scheinbaren Widerspruch zur konventionellen Symmetrieanalyse des magnetoelektrischen Effekts, welcher in diesen Systemen eigentlich aus Symmetriegründen verboten wäre.
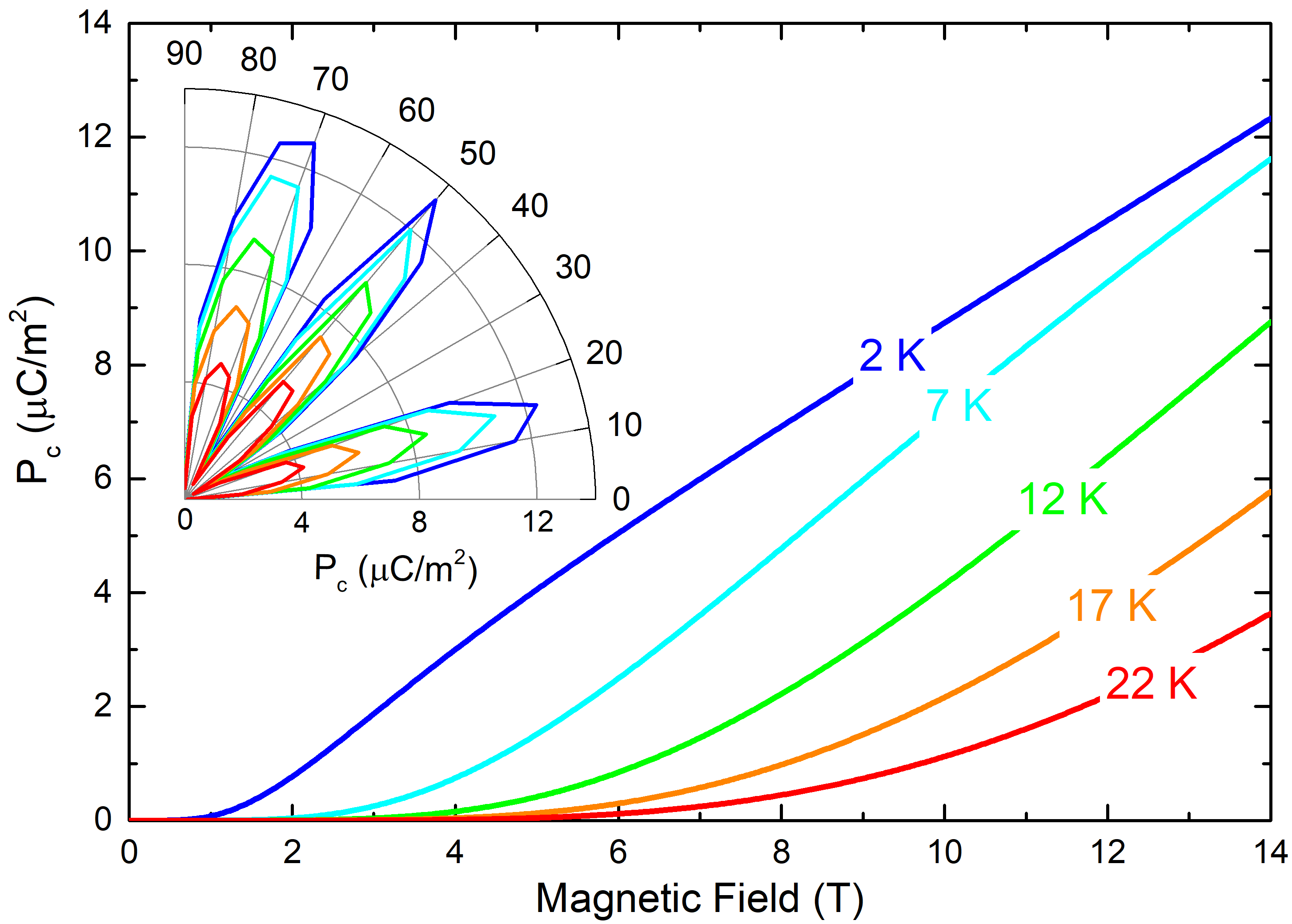
Ivan Chemakin: Kunst im Labor
Kunst im Labor – ein Katalog zum Projekt umfasst alle Arbeiten, welche in Rahmen von Kunstprojekt in unseren Laboratorien entstanden sind.
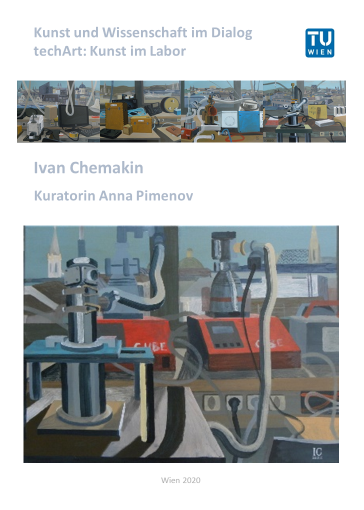
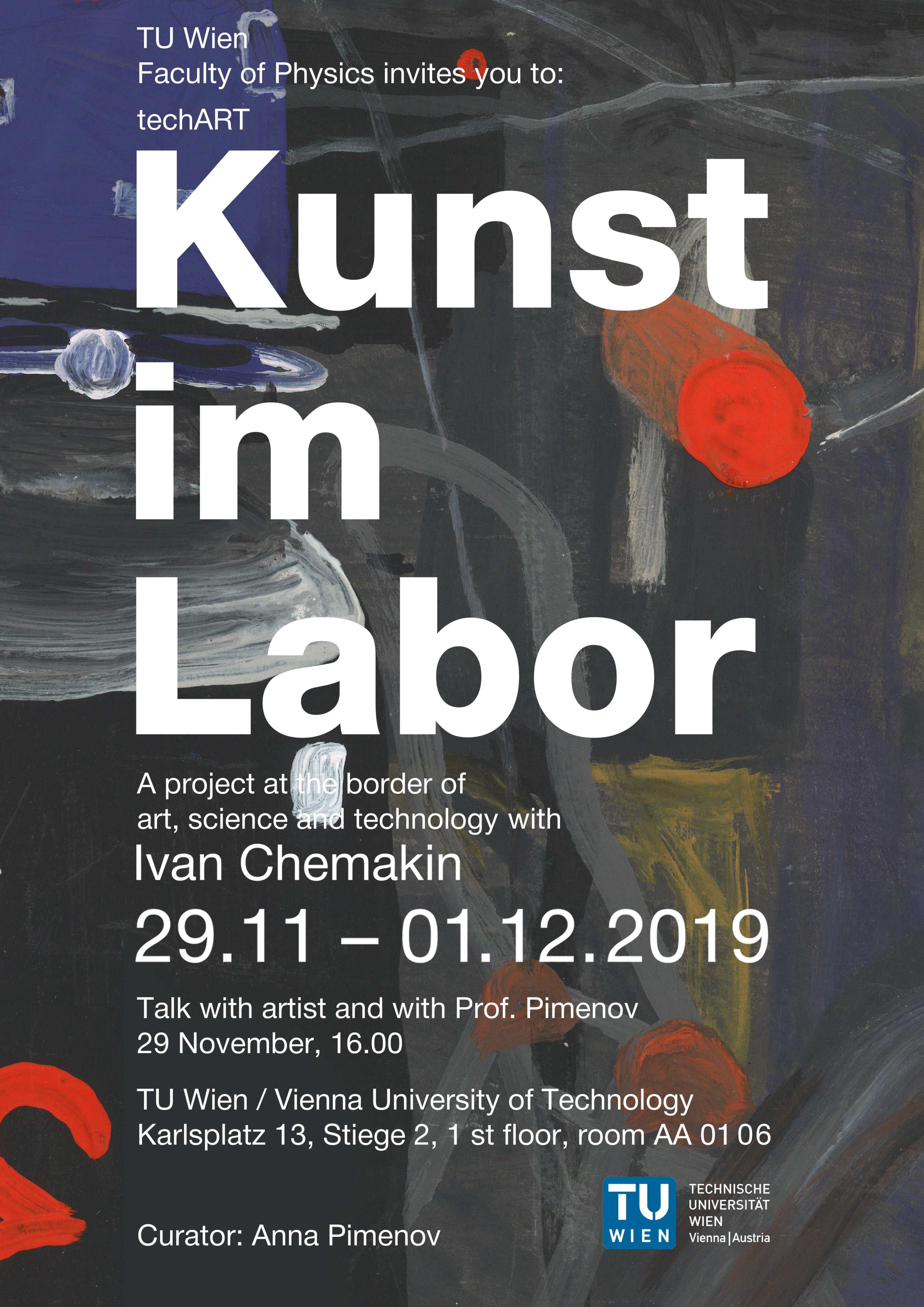
Controlling Light by Manipulating Electromagnons
Within some specialised materials, light can appear to move in mysterious ways, rotating around its direction of propagation, and becoming dimmed to different degrees, depending on its direction of travel. Professor Andrei Pimenov and his team at the Vienna University of Technology investigate how these properties are linked to recently discovered quasiparticles named ‘electromagnons’. The team’s work could soon open up new ways to consciously control the propagation of light within these unique solid materials, with numerous exciting technological applications.
(article)We welcome Janek Wettstein
Janek studied physics at Karlsruhe Institute of Technology from 2013 to 2019 where he succesfully performed his master thesis in the Heisenberg Spin-dynamics Group of PD Khalil Zakeri. In his thesis he investigated collective excitations in one monolayer FeSe grown on SrTiO3 using spin-polarized electron energy loss spectroscopy. In July 2019 Janek joined the group as a doctoral candidate.
New Project
EMERGE: Emergent Electrodynamics in Frustrated Magnets
One fascinating consequence of the constrained spin degrees of freedom in certain frustrated magnets is the ability to describe their collective behaviour by emergent gauge fields. Interestingly, this emergent description can be shown to express a novel form electrodynamics on top of the collective magnetic behaviour where new particles such as magnetic monopoles can exist. This project aims to study this emergent behaviour by probing these novel excitations and their dynamics using terahertz radiation.
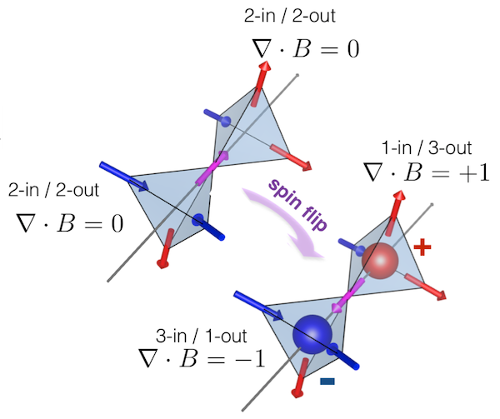
The perfect terahertz beam - thanks to the 3D printer
TU Wien has succeeded in shaping terahertz beams with extremely high precision. All that is needed for this is a simple plastic screen from a 3D printer.
Terahertz radiation can be used for a wide variety of applications and is used today for airport security checks just as much as it is for material analysis in the lab. The wavelength of this radiation is in the millimeter range, meaning that it is significantly larger than the wavelength of visible light. It also requires specialized techniques to manipulate the beams and get them into the right shape. At TU Wien, shaping terahertz beams is now something of a resounding success: with the help of a precisely calculated plastic screen produced on the 3D printer, terahertz beams can be shaped as desired.
(read more) (article)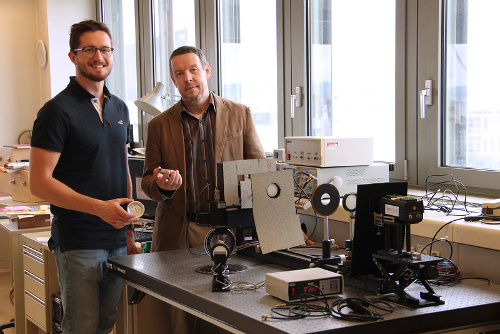
Successful PhD Thesis Defense
Congratulations to our group member and friend Uladzislau Dziom for successfully defending his PhD thesis, "THz spectroscopy of novel spin and quantum Hall systems", supervised by Prof. Dr. A. Pimenov.
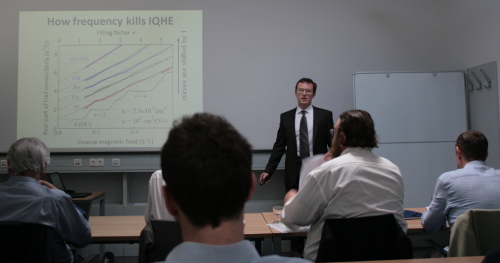
Using electricity to switch magnetism
At TU Wien, a major step has been taken towards linking electrical and magnetic material properties, which is crucial for possible applications in electronics.
It’s not exactly a new revelation that electricity and magnetism are closely linked. And yet, magnetic and electrical effects have been studied separately for some time now within the field of materials science. Magnetic fields will usually be used to influence magnetic material properties, whilst electrical properties come down to electrical voltage. Then we have multiferroics – a special group of materials that combine the two. In a new development, TU Wien has managed to use electrical fields to control the magnetic oscillations of certain ferrous materials. This has opened up huge potential for computer technology applications, as data is currently transferred in the form of electrical signals but stored magnetically.
(read more) (article)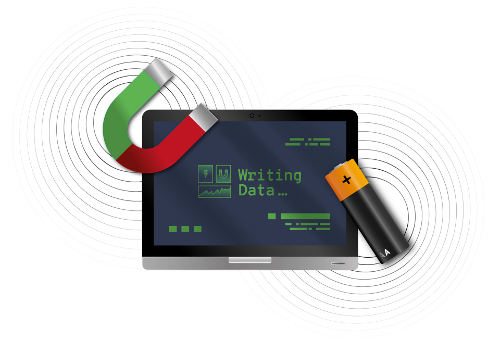
3rd Grandmaster PhD Workshop in Physics, 2018 TU Wien
Following the success of previous workshops organized in Augsburg and Budapest, we are pleased to announce the 3rd Grandmaster PhD Workshop in Physics. This year the workshop will be held at TU Wien, from February 19 to 23.
The workshop aims to provide a forum for young scientists to present their results that are of interest to a scientific community, yet in a colloquial atmosphere. MSc, PhD student and post-doc participants can contribute either an oral (25 minutes talk + 5 minutes for discussions) or poster presentation. Through this, the participants can get insight into on-going research projects in the field of solid state physics, and become familiar with related experimental techniques at other contributors’ institutes. In the long term, we hope that this workshop can help initiate bilateral or multilateral research programs.
The highlight of the workshop will be a one-day-symposium entitled From Topology to Superconductivity. Distinquished experts in the field will give tutorial talks covering both the fundamental aspects and potential applications.
We welcome Nadezhda Kostyuchenko
Nadezhda defended his PhD at the Moscow Institute of Physics and Technology (State University), Russia in 2016. In her thesis she studied the influence of the crystal-field and exchange interactions on properties of rare-earth magnetics: iron borates, aluminum borates and intermetallics. Nadezhda joins our group to continuing her research on the theory (analytical and numerical) of magnetic, magnetoelecric and others properties of multiferroics, magnetoelectrics and intermetallics.
We welcome Dávid Szaller
Dávid defended his PhD at the Budapest University of Technology and Economics, Hungary in 2017. In his thesis he studied the low energy optical properties of magnetoelectric multiferroic crystals. One of the most fascinating effect these materials present is directional dichroism, i.e. different absorption of counter-propagating light beams, which David has observed in several multiferroics in the THz frequency regime. Besides the optical experiments, in order to gain a deeper insight into this phenomenon he also carried out group-theoretical and statistical-physical analyses. David joins our group as a PostDoc, continuing his research on the low frequency excitations of multiferroics.
Measured for the first time: direction of light waves changed by quantum effect
The 'quantized magneto-electric effect' has been demonstrated for the first time in topological insulators at TU Wien, which is set to open up new and highly accurate methods of measurement.
A light wave sent through empty space always oscillates in the same direction. However, certain materials can be used to rotate the direction in which the light is oscillating when placed in a magnetic field. This is known as a 'magneto-optical' effect.
After much speculation spanning a long period of time, one variant of this type of effect has now been demonstrated at TU Wien for the first time. Rather than switching the direction of the light wave continually, special materials called 'topological insulators' do so in quantum steps in clearly defined portions. The extent of these quantum steps depends solely on fundamental physical parameters, such as the fine-structure constant. It may soon be possible to measure this constant even more accurately using optical techniques than is currently possible via other methods. The latest findings have now been revealed in the open-access journal 'Nature Communications'.
(read more) (article)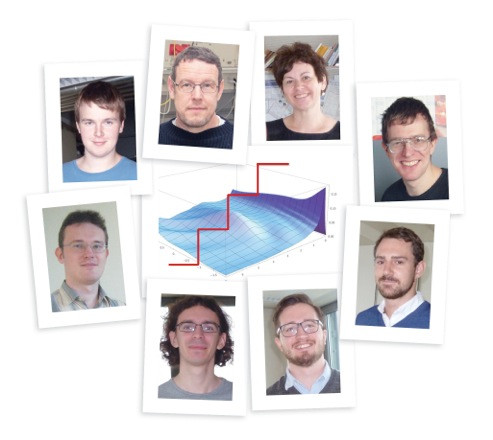
We welcome Lorenz Bergen
Lorenz studied Physics at TU Wien from 2010 to 2016, completed with a Diploma thesis performed in the group of Prof. Aumayr. There he investigated the nanostructuring of surfaces by swift heavy ion impacts. After working at the Helmholtz-Zentrum Dresden-Rossendorf, Lorenz came back to Vienna in 2017 and joined the group as a PhD-Student.
We welcome Evan Constable
Evan is an Australian researcher with a background in terahertz spectroscopy. In 2014, he completed his PhD at the University of Wollongong, Australia where he focused on spectroscopy of magnetic materials. During his studies, he developed a keen interest into the complementary nature of terahertz and neutron spectroscopic techniques. This interest brought him to Grenoble, France, where he undertook a postdoc position with the CNRS at Institut Néel from 2015 to 2017. Working closely with researchers at the SOLEIL synchrotron and ILL reactor, Evan continued to develop projects that brought together complementary terahertz and neutron scattering experiments on frustrated magnetic systems. Evan now joins the solid-state spectroscopy group as a postdoctoral researcher. His primary research interests remain in the fields of terahertz spectroscopy, frustrated magnetism and multiferroics.
We welcome Jan Gospodarič
Jan joined the group in the beginning of 2017 as a doctoral candidate. He previously studied physics at University of Ljubljana, Slovenia from 2010 to 2016, a study he ended with a successful Master's thesis project at the Jožef Stefan Institute in a research group of prof. Dragan Mihailović. His thesis examined the optical spectroscopy of a hidden state in 1T-TaS2 in visible regime.
We welcome Lukas Weymann
Lukas studied from 2011 to 2016 physics at the Leibniz University in Hannover. He wrote his master thesis at the Max Planck Institute for Gravitational Physics (Albert Einstein Institute) in the independent research group of Dr. Holger Pletsch. His thesis was based on the gamma-ray timing of an extreme black widow binary pulsar.
New PostDoc position
There is a new PostDoc position available in the area of optical and infrared spectroscopy.
Successful PhD Thesis Defense
Congratulations to our group member and friend Markus Schiebl for successfully defending his PhD thesis, "Dielectric spectroscopy at the spin-driven ferroelectric phase transition in chiral multiferroic DyMnO3", supervised by Prof. Dr. A. Pimenov.
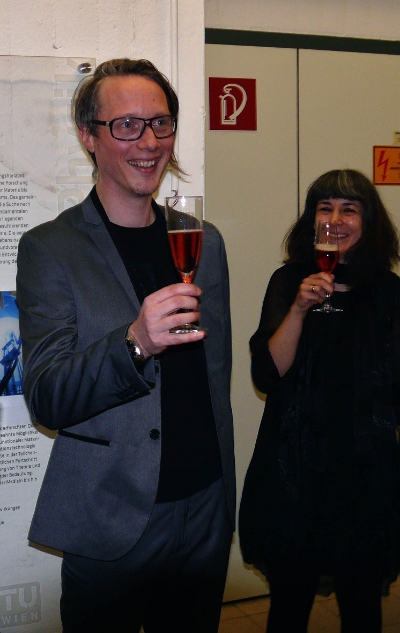