How do good metals turn ‘bad’?
This research article published in Nature Communications has been featured in a press release of TU Wien (English version) and in Physics World, as well as in Austria's leading newspapers (Der Standard) and other public media.
Although we daily utilize them, we rarely pose the question about what makes a metal metallic. Text books cite the good electrical conductivity, malleability and their shiny appearance, but these properties are found together only in elements like silver or aluminum. For instance, some alloys are hard and brittle, while transparent metals like indium tin oxide (ITO; nowadays used in essentially all display applications) appear by no means ‘shiny’ because, by definition, they do not reflect visible light. In the latter case, high reflectivity is still found, but at smaller frequencies in the infrared – well below the ‘visible’ range seen by the human eye.
Condensed-matter science defines metals as materials with itinerant electrons, which carry electrical current in solids. Here, the electrical conductivity increases when reducing the temperature because scattering of electrons (with other electrons or with the lattice) diminishes upon cooling. From the theoretical point of view, there is an upper limit to the scattering rate: in a typical (let us call it a ‘good’) metal an electron can be scattered once per atom at most, since there is nothing in between that it could collide with. Indeed, the conductivity approaches this minimum value, known as ‘Ioffe-Regel-Mott’ limit (IRM limit), in many metals and does not decrease below that.
Our research group is interested in materials that seem to violate the IRM limit. The group leader, Andrej Pustogow, states that the focus is on “compounds that cannot be described by the established conventional concepts of solid-state physics”. The present work has been performed on millimeter-sized single crystals that have been synthetically grown by chemists. Already a minuscule modification of the stoichiometry can have dramatic effects on the transport properties and even change the behavior from metallic to insulating. While metals are good conductors, electrical current cannot flow in insulators which makes them fully transparent to low-frequency electromagnetic radiation. Similar to glass or water, which are transparent in the range of visible light, the insulators studied here are transparent in the infrared range or at even lower frequencies.
Together with his international collaborators Vladimir Dobrosavljević (Florida State University), Martin Dressel (University of Stuttgart) and Simone Fratini (Universite Genoble Alpes), Andrej Pustogow studied the optical and electrical properties of organic crystals while their behavior changed from metallic to insulating. “We observe metallic behavior with a conductivity that is a factor 100 to 1000 lower than the IRM limit”, says Pustogow. The major question arising is whether this is an entirely different type of metal requiring a theoretical framework different from the quasiparticle description in terms of Landau’s Fermi-liquid theory. Similar ‘bad metallic’ behavior has been reported also in many other correlated electron systems.
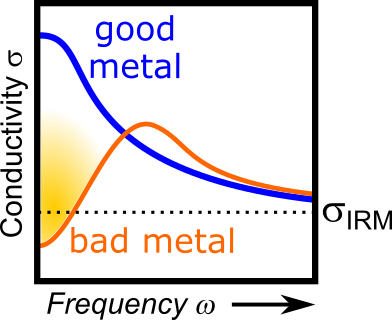
“Our results clearly show that ‘bad metals’ are essentially ‘good’ at high frequencies, in accord with Landau’s quasiparticle framework” says Pustogow. “Now it remains to elucidate the localization mechanisms at low frequencies.” One possibility is weak disorder, which can emerge from tiny amounts of imperfections in the crystal, such as defects or stacking faults, causing localized regions of the crystal to ‘drop out’ from the metal and thus act essentially as vacancies. This way, the motion of conduction electrons is hindered as they get stuck in such a ‘non-metallic’ area. The probability of getting stuck is higher at low frequencies because the electrons travel large distances through the crystal during a period of the electromagnetic field. At high frequencies electrons are less likely to hit such a region as they only move over shorter distances before the polarity of the electric field switches.
The present results demonstrate the importance and necessity of optical spectroscopy for clarification of the most relevant unsolved research questions of condensed-matter science. Certainly, many observations that were claimed to require a novel description beyond Landau’s quasiparticle framework can be still explained in terms of Fermi-liquid theory, provided it is properly amended. The understanding of metallic behavior in strongly correlated electron systems is particularly important for unconventional superconductivity, which has been discovered more than 40 years ago, but is not fully understood until today. The new results will provide useful guidance leading the scientific community to exciting findings in the near future.
Original article:
A. Pustogow, Y. Saito, A. Löhle, M. Sanz Alonso, A. Kawamoto, V. Dobrosavljević, M. Dressel, and S. Fratini
Nat. Commun. 12, 1571 (2021),
DOI:10.1038/s41467-021-21741-z, arXiv:2101.07201.