Impurity states realize the 'Best Thermoelectric'
This research article published in Nature Communications has been featured in a press release of TU Wien (English version), ELE Times and other media.
Thermoelectrics are considered a high-potential future technology as they allow the direct interconversion of thermal and electrical energy, which can be useful for manifold applications from energy harvesting to refrigeration. Discovered more than 200 years ago in 1821 by German physicist Thomas Johann Seebeck, nowadays, thermoelectricity spans a wide, inter-disciplinary field of theoretical and experimental research activities of physicists, chemists and materials scientists searching for new materials with high thermoelectric performance. The relevant quantity reflecting the conversion efficiency is the dimensionless figure of merit ZT. In order to achieve high ZT values at a given temperature T, large thermopower S and electrical conductivity σ, but low thermal conductivity κ are required: ZT = S2σT/κ. Due to the interdependence of all these physical properties for charge and heat transport, it turned out an extremely tough challenge to increase the absolute value of ZT.
So far, milestones and novel strategies have mostly focused on working towards a ‘phonon glass electron crystal’ concept by reducing the crystal lattice contribution to the thermal conductivity κph as it is somewhat decoupled from the other electronic parameters. On the other hand, comparably little emphasis has been placed on finding optimization strategies for the electronic part of ZT. Thus, success has been limited by the complexity of the problem and lack of innovative strategies. Only a small, confined fraction of materials classes – i.e. narrow-gap semiconductors with high band degeneracy and large number of pockets on the Fermi surface – have been perceived as viable thermoelectric candidates. Here, we show an original and straightforward approach that could open an array of possibilities, enabling different types of materials to become suitable thermoelectrics.
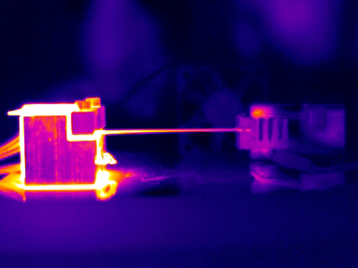
New strategy to enhance the thermoelectric properties
In 1996, Mahan and Sofo employed a mathematical concept of a singularity in the electronic transport continuum, hoping to answer the simple but fundamental question: ”What electronic structure provides the largest ZT?”. They found that the ideal thermoelectric material would have electrons contributing to transport only in an infinitely narrow energy interval. In other words, conduction electrons should exist just within a small distribution of energies, or in the optimum case, at a single energy value. Their paper [Proc. Natl Acad. Sci. 93, 7436–7439 (1996)], now cited over 1700 times, is well known and taught to students right away. While their mathematical calculations have been somewhat discarded as an idealized picture, we propose that it is indeed possible to revert to this original idea, which we put into practice in a real material.We recognize that the Anderson transition in an electronic impurity band is a direct realization of Mahan and Sofo’s original concept. Anderson and Mott, both sharing the nobel prize in 1977, discovered that when randomly adding impurity atoms to a clean system, for instance dopants in a semiconductor, their electrons are localized. Mott’s original idea was that electrons are trapped in the attractive Coulomb potential of the impurity ions, which becomes screened at a sufficiently high density of impurity atoms, or if the distance between the atoms decreases below a threshold value. However, soon it was shown by Anderson that also disorder, i.e. the random distribution of the impurities itself, is a relevant effect and causes localization in terms of an exponential decay of the electron wave functions at the impurity sites due to the multiple scattering and destructive interference of the charge carriers. Upon continuously adding more and more impurity electrons, they remain localized until a percolation threshold value, known as the Anderson transition, where a singularity in the transport function occurs – the condition of Mahan and Sofo’s ‘best thermoelectric’.
We realized such a scenario in the ternary Heusler alloy Fe2VAl using a simple yet innovative approach: thermal quenching directly tunes the degree of disorder and impurities within a single sample. At high temperatures, thermal excitations lead to the formation of anti-site defects and disorder in this material. Consequently, the electronic and magnetic properties change dramatically, yielding the Anderson insulator-metal transition and emergence of magnetism. By continuously tuning the degree of anti-site disorder in Fe2VAl we were able to drive the system across the Anderson transition and found a surprising enhancement of the thermoelectric performance by more than an order of magnitude (simply by changing the heat treatment of the same sample). Since disorder and impurity bands are ubiquitous, our universal approach can possibly be employed in many materials, providing a novel route for present and future thermoelectric research to improve the electronic part of ZT.
Beyond the field of thermoelectrics, our work reignites the interest in impurity conduction, disorder and Anderson-localization as fundamental puzzles in condensed matter physics.
Original article:
F. Garmroudi, M. Parzer, A. Riss, A. V. Ruban, S. Khmelevskyi, M. Reticcioli, M. Knopf, H. Michor, A. Pustogow, T. Mori, and E. Bauer
Nat. Commun. 13, 3599 (2022),
DOI:10.1038/s41467-022-31159-w.